Cosmic rays in molecular gas
Galactic cosmic rays (CRs) are a ubiquitous source of ionization and heating of the interstellar gas. In dense astrophysical environments, such as molecular clouds and pre-stellar cores (where UV and x-ray photons are extinguished), the ionization and heating are completely dominated by CRs. One of the principal aims of the CAS-Theory group is to understand the physics of low-energy CRs in molecular gas, by combining advanced methods of the kinetic theory and plasma physics and applying available observational constraints.
Below we highlight several topics, representing our current research on theory of CRs in dense gas as well as on the key physical and chemical processes governed by CRs in such environments.
Theory of CR penetration into dense gas
Diffusive vs. ballistic propagation of CRs: The penetration of Galactic CRs into molecular clouds and their further transport in dense gas is a complex physical phenomenon. Depending on a number of conditions, CRs can either move freely (“ballistically”) along the local magnetic field lines, or experience significant scattering on small-scale field fluctuations and propagate diffusively. Also, CRs lose energy due to ionizing collisions with gas molecules. In combination with the realized transport regime, this shapes the local energy spectrum of CRs and thus sets the local ionization rate ζ – the chief parameter that controls a variety of physical and chemical processes. Given that different transport regimes result in quite different dependencies of ζ on gas column density N, the CR transport could be constrained from available and future observations. We have derived analytical expressions for ζ vs. N, generally applicable to describe the diffusive or ballistic regimes of CR propagation (Silsbee et al. 2019). The results are illustrated in Figure 1. Observational data suggest a relatively steeply decreasing ζ(N) in molecular cloud envelopes, which is matched better with the model of diffusive propagation. While the currently available data are not precise enough for drawing solid conclusions, our theoretical work provides the essential basis for the analysis of upcoming data – which will eventually allow us to identify the dominant regime of CR transport in molecular clouds.
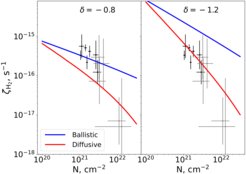
Figure 1: Primary ionization rate ζH2 of molecular hydrogen as a function of column density of hydrogen atoms N. The solid blue and red lines depict theoretical predictions assuming, respectively, pure ballistic and pure diffusive regimes of CR propagation. The two panels demonstrate results for two model values of the spectral slope δ of nonrelativistic interstellar protons (which completely dominate CR ionization in the shown cases). The points and error bars represent observations (Neufeld & Wolfire 2017), with black points showing the cases where the H2 column density has been measured directly.
Secondary CR ionization: CRs interacting with the gas generate electron-ion pairs, with electrons having sufficient energy to produce further ionization. These processes of primary and secondary ionization are characterized by the respective ionization rates, ζp and ζsec. While ζp can be straightforwardly derived for a given CR spectrum, computing ζsec is a much more complex problem. Recently, we have rigorously calculated the energy spectrum of electrons that are produced by interstellar CRs penetrating dense molecular gas (Ivlev et al. 2021). Figure 2 illustrates our findings, showing the derived dependence of ζsec/ζp on N. We see that this ratio steadily increases with gas column density. This behavior is quite different from the assumption of a constant ζsec/ζp, traditionally accepted in the literature. Knowing the exact spectrum of secondary electrons makes it possible to accurately evaluate characteristics of various important processes driven by CRs in molecular gas, such as the local rates of gas heating and H2 dissociation, the local magnitude of the UV field (due to H2 fluorescence), generation of x-ray emission as well as of IR emission (due to H2 rovibrational transitions), etc.
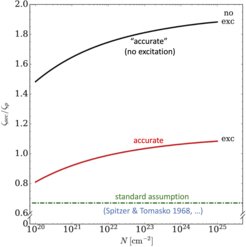
Figure 2: Ratio of the secondary to primary ionization rates of H2 as a function of the gas column density. The red curve (“exc”) shows the exact case where excitation collisions with H2 are included. For methodological reasons, we also plot the model case of no excitation (“no exc”, illustrating the importance of excitation energy losses compared to ionization losses). The horizontal dashed–dotted line indicates the “standard” value of ζsec/ζp often adopted in the literature.
Thermal damping of small-scale compressive turbulence: The presence of MHD turbulence at scales of an AU or less can lead to efficient scattering of the sub-GeV CRs, and thus may reduce their penetration into molecular clouds. Furthermore, such turbulence is likely to be the dominant source of relative motion between dust grains, determining their coagulation in dense gas. Hence, understanding the turbulent cascade at small scales is important for a number of astrophysical applications, especially in molecular clouds. We have identified a generic mechanism for the thermal damping of compressive waves in the interstellar medium, occurring due to radiative cooling of gas, and studied the impact of this damping on weak magnetosonic turbulence (Silsbee et al. 2021a). Figure 3 shows the steady-state turbulent spectrum calculated for the Kolmogorov cascade in the cold neutral medium (CNM) and in a typical molecular cloud envelope. It is evident that the thermal damping has a profound effect on the turbulent spectrum, and that this effect is extremely sensitive to the magnitude of the magnetic field. The cutoff wavelength for magnetosonic turbulence in molecular clouds becomes tens to hundreds of times larger when the thermal damping is added to the regular damping mechanisms, implying that more CRs are able to freely penetrate into dense molecular gas.
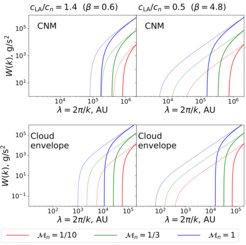
Figure 3: The energy spectrum of magnetosonic turbulence W(k), calculated assuming a Kolmogorov cascade. Thermal damping is included for the solid lines, but omitted for the dotted lines. Different colors correspond to different injection velocities v0, as shown in the legend in terms of the sonic Mach number Mn = v0/cn (cn is the sound speed in a neutral gas). Turbulence is injected at a scale of λ0 = 1 pc for the cloud envelope, and λ0 = 10 pc for the CNM. In the left-hand panels, the magnetic field is chosen such that the speed of loaded Alfven waves is cLA = 1.4cn (plasma β = 0.6), while in the right-hand panels cLA = 0.5cn (plasma β = 4.8).
Implications for physics and chemistry in clouds and disks
Icy mantles on dust grains: Dust grains in cold pre-stellar cores can grow icy mantles, where abundant gas-phase species can freeze out. Existing gas-grain chemical models predict formation of thick icy mantles of a few hundred monolayers around ~0.1 μm dust particles, containing mainly water and CO. Collisions of CRs with grains are one of the main physical mechanisms that control the structure and composition of icy mantles at different evolutionary stages of the cores. We have computed the desorption rate of icy mantles as a function of the size and composition of both the grain and the mantle (Silsbee et al. 2021b). Combining existing models of CR-induced desorption with our models of CR transport allowed us to accurately calculate the mantle growth in dark regions of molecular clouds. We showed that different desorption mechanisms dominate for grains of different sizes and in different regions of the cloud, leaving some grains free of volatile material, as illustrated in Figure 4.
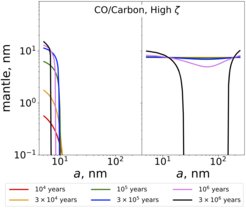
Figure 4: Thickness of icy mantles as a function of grain size, assuming an MRN size distribution and a ballistic penetration of CRs into a molecular cloud core. All mantle material is in the gas phase at time t = 0, different color curves correspond to different evolutionary times. The left-hand and right-hand panels correspond to an outer (n~104 cm–3) and inner (n~106 cm–3) core region, respectively. The composition of the mantle and the grain (CO ice on amorphous carbon material) is labelled on the panels.
CR processing of ice: A large body of experimental work has shown that the interaction between CRs and ices similar to those coating interstellar grains can result in the efficient production and desorption of various chemical species observed in cold ISM regions. Yet, understanding of these phenomena critically depends on the knowledge of microphysical processes occurring in ices upon the impact of individual CRs. We have shown that CR tracks in amorphous solid water can be treated statistically as a cylindrical core with an average radius that is a function of the initial CR energy (Shingledecker et al. 2020). Interactions between energetic ions and ice targets were simulated using a Monte Carlo code, which allowed us to follow secondary electrons down to sub-excitation energies in the material, as depicted in Figure 5. We found the radius of the cylindrical core (determined by the average stopping range of secondary electrons) to be as large as ~10 nm, substantially larger than the value often assumed in astrochemical models.
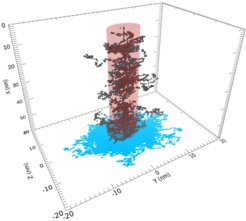