How to spin a disk around young protostars
How do stars and planets form? Scientists are now one step closer to pinning down the conditions for the formation of proto-stellar disks. Observations of three systems in the early stages of star formation in the Perseus cloud revealed that the profile of the angular momentum in these systems is between that expected for a solid body and pure turbulence, indicating that the influence of the core extends further out than previously thought. These findings could lead to more realistic initial conditions for numerical simulations of disk formation.
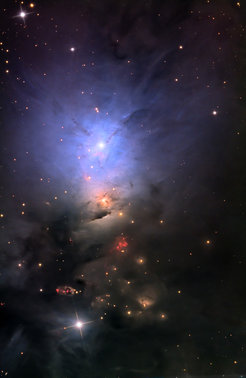
The main steps of star and planet formation are well understood: a dense, interstellar cloud will collapse under its own gravity; a central core forms as well as a proto-stellar disk due to the conservation of angular momentum; finally, after about 100,000 years or so, the star will become dense enough to ignite nuclear fusion at its centre and so will start to shine, while in the disk, planets will form. But there are still many open questions about the details of this process, e.g. what is the role of angular momentum in disk formation or how does the circum-stellar disk gather most of its mass?
An international team of scientists led by the Max Planck Institute for Extraterrestrial Physics (MPE) has now observed three of the youngest proto-stellar sources in the Perseus molecular cloud. These sources are close to edge-on in the plane of the sky, allowing a study of the velocity distribution of the dense cloud.
“This is the first time that we were able to analyze the gas kinematics around three circum-stellar disks in early stages of their formation,” states Jaime Pineda, who led the study at MPE. “All systems can be fit with the same model, which gave us the first hint that the dense clouds do not rotate as solid body.” A solid body rotation is the simplest assumption, which describes the gas in the dense cloud with a fixed angular speed at any given radius. The model best describing all three systems is in between those expected for solid body rotation and pure turbulence.
Furthermore, when comparing these observations to previous numerical models, it is clear that magnetic fields play a role in the formation of these disks: “If a magnetic field is included it makes sure that the collapse is not too fast and the gas rotation matches the observed one,” explains Pineda. “Our latest observations give us an upper limit on the disk sizes, which are in great agreement with previous studies.”

In particular, the specific angular momentum of the in-falling material is directly related to the possible maximum Keplerian radius of the proto-stellar disk. Assuming a stellar mass of about 5% of the mass of our Sun, the scientists estimate that the upper limit of the Keplerian disk is about 60 Astronomical Units, or about the double the size of our planetary system, in agreement with previous estimates. This suggests that big disks (greater than 80 AU) cannot be formed early in the life of a star, and therefore affects the starting point for planet forming scenarios.
The next step for the astronomers will be to observe such systems at various stages in their evolution and in different environments to check if these influence the specific angular momentum profile. These findings can then be incorporated in or compared with numerical simulations to better understand the co-evolution of the dense core forming a star and the circum-stellar disk forming planets.