Gamma rays reveal pair plasma from a flaring black hole binary system
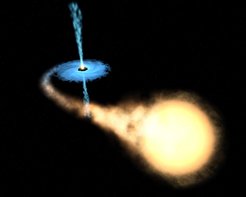
Artist's impression of a microquasar with a stellar black hole, accreting matter from a companion star, forming an accretion disk and jet outflows.
Black holes are a prominent area of research throughout astrophysics, and the disappearance of matter behind its event horizon is fascinating. In a microquasar, the black hole typically has a mass of a few times that of our Sun and is accreting matter from a nearby companion star. The matter flow forms an accretion disk around the black hole, which is heated to X-ray temperatures, and occults the inner region where matter disappears behind the event horizon. In the accretion process, huge amounts of energy are released as the material falls into the gravitational well of the black hole, somehow heating the surrounding gas and ejecting hot plasma jets with nearly the speed of light. Most of the time a microquasar is rather quiet and only swallows matter slowly and steadily from the inner edge of the accretion disk around the black hole. In these quiescence phases and even more so during occasional flares, the inner region near the black hole is opaque even for high-energy gamma rays. Thus it remained a challenge to study how the accretion of matter actually results in the observed output of energy.
Microquasars, such as V404 Cygni, reside also in our own Galaxy, hence they are “nearby” by astronomical standards, allowing the astronomers to study them in detail. V404 Cygni is one of the closest of its kind, located only 8000 light-years away in the constellation Cygnus, the Swan, and the parameters of this binary system are well known. After 26 years of quiescence, it turned into a flaring state in summer 2015. From 17 to 30 June 2015, the astronomers observed intense X-ray and gamma-ray emission, many times brighter than the Crab Nebula, which is the brightest persistent source in the high-energy sky at normal times. V404 is a peculiar source, as its multi-wavelength properties suggest that the strong jets possibly point towards us. “Only INTEGRAL with its exquisite instrumental payload permit such findings," says Jerome Rodriguez from CEA Paris, co-author of the paper and author of a multi-wavelength paper on V404 Cygni.
V404 Cygni gamma-ray observations
This animation shows the observations with INTEGRAL (left) and the corresponding energy output of the source V404 Cygni (right) across the X-ray band. This bright emission is attributed to the hot material in the accretion disk, from its spectral distribution; its flickering reflects instabilities in the accretion flow. The other two bright locations in the field of view are unrelated known sources in the constellation Cygnus.
“Such an extremely powerful outburst should lead to the creation of substantial amounts of matter in the form of pair plasma, i.e. matter and anti-matter particles, according to Einstein’s formula E=mc^2” explains Roland Diehl, lead researcher from the Max Planck Institute for Extraterrestrial Physics (MPE). “Many of these particles should therefore annihilate with each other, creating their very characteristic high-energy signature, with a gamma-ray line at rest energy 511 keV. And exactly this line, with expected kinematic distortions, we were able to observe. This is the first time that we see a clear positron signal from a well-known black-hole binary!”
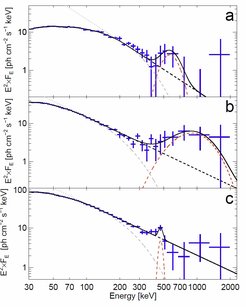
Spectral distribution of the signal above 30 keV during the 3 epochs, which correspond to days 1-2, 3-4.5, and 8-9 in the above animation. For each epoch, there is a clear excess of radiation above an energy of 500 keV. This signal can be explained as a combination of thermal radiation from the accretion disk (light grey dashed line) and the annihilation from the pair plasma produced near the black hole (red dashed line).
The data were grouped into three epochs of about 3 days each, and a highly significant excess at MeV energies was detected in each epoch. Theoretical work showed that this signature cannot come from the accretion disk nor corona, and can only be explained by the production of electrons and positrons. These are created in sufficient abundance from the high-energy gamma-rays near the black hole during intensified accretion. This process is very efficient due to the small size of the source (less than 100 km or 3-10 times the gravitational radius of the black hole). The pair plasma is continually created in this regionand annihilated on its way out, still relatively near to the black hole. The signal observed during epoch 3 is somewhat puzzling, as it could even be from the annihilation of positronium atoms, i.e. an exotic atom formed from a positron representing the atomic nucleus and an electron. Such annihilation is well observed with INTEGRAL throughout the Galaxy by the Max Planck scientists, yet occurs typically in much colder and less dense environments.
“When the X-ray flaring of V404 Cygni faded, the annihilation signal also vanished,” says Thomas Siegert from MPE, lead author of the paper describing the observations in the article in ‘Nature’. “This measurement gives us information from the inner accretion disk, from processes at the immediate surroundings of the black hole. Our analysis naturally connects the process of pair production to the plasma flow later observed in radio jets and its lobes, which are found much further away from the inner source.”
Pair plasma is easily accelerated and attains velocities of half the speed of light, as observed in radio emission. This outflow of pairs also makes microquasars efficient factories of antimatter, enriching the surrounding medium with positrons. They were listed as candidate sources to lead to the large-scale diffuse glow of the entire Galaxy in annihilation gamma rays, with its puzzling brightness distribution on the sky. These results shed new light on positron emission from microquasars, and can help to understand why diffuse annihilation radiation is so bright towards the bulge region of our Milky Way.
Note:
Microquasars are named after their much more massive counterparts, “quasars” or quasi-stellar objects. These are very distant but at the same time very bright galaxies, which harbour a supermassive black hole at their centre. These black holes are “active”, i.e. they are accreting and swallowing matter from their surroundings. The infalling matter generates vast amounts of energy, making quasars extremely powerful sources in the very distant universe.