Science
MICADO will address a large number of science topics that span key elements of modern astrophysics. Focusing on its primary capabilities of sensitivity and resolution, the science drivers for the design of MICADO concentrate on the following main themes: (i) galaxy evolution via observations of galaxies in situ at high redshift as well as relic populations in local galaxies, (ii) the Galactic Center and supermassive black holes in galaxy nuclei, (iii) intermediate-mass black holes in stellar clusters, (iv) characterization of exoplanets and circumnuclear disks at small angular scales, and (v) the solar system. A few of these are highlighted below.
In Situ Galaxy Formation and Evolution
We now have a fairly robust outline of the cosmic evolution of global galaxy properties, and hence the first pieces of evidence about how galaxies assembled and transformed into the present day Hubble sequence. An obvious next step is to resolve the faint distant galaxies on sufficiently small scales to assess their subgalactic components, including disk structures, nascent bulges, clumps, and globular cluster progenitors. The current view is limited by spatial resolution, which corresponds to ~ 1 kiloparsec in the best cases (space-based telescopes or adaptive optics on 8 m class ground-based telescopes). In particular, relatively unexplored regimes include lower-mass galaxies, comprising the bulk (by number) of the galaxy population, and galaxies at early cosmic times, when they were building their first stars. Figure 1 illustrates the type of detailed structure within high-redshift galaxies that MICADO might be able to detect.
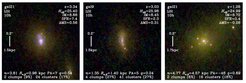
Relic Stellar Populations
An alternative probe of galaxy evolution is the relic populations in local galaxies, in which we use photometry of individual stars to generate a color magnitude diagram (CMD). The various features of a CMD relate to stars formed at different cosmic times. In particular, stars on the horizontal branch enable scientists to trace the star formation history of galaxies to z > 6, to the reionization epoch. The ultimate goal is to probe the central regions of elliptical galaxies in the Virgo Cluster. The high surface brightness, due to extreme stellar crowding, makes this very challenging. The James Webb Space Telescope (JWST) will only be able to analyze the outskirts of these galaxies, while the higher resolution of MICADO will enable it to reach almost to the center, where the bulk of the stars can be found.
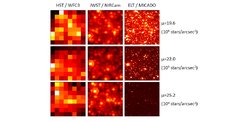
The Galactic Center
One of the obvious rationales for astrometry is using stellar proper motions to probe the existence and masses of black holes in galactic nuclei, stellar clusters, and nearby low-mass dwarf galaxies.
The Galactic Center is a unique laboratory for exploring strong gravity around the closest massive black hole, as recently demonstrated by the remarkable results from GRAVITY and the award of the Nobel Prize for Physics in 2020. The fundamental goal is to measure the gravitational potential in the relativistic regime close to the central black hole via stellar motions, using very faint stars that are likely to be present but cannot be detected nor studied with any other facility prior to the ELT. These motions may also reveal the theoretically predicted extended mass distribution from stellar black holes that should dominate the inner region, as well as test for a distributed component of dark matter. An exciting opportunity lies in measuring the spin of the black hole, a goal that is more tractable via spectroscopy than astrometry. This can be achieved by tracking a late-type star whose complete orbit lies within ~ 10 milliarcseconds (0.5 light-days, about 1/10 of the S2 orbit) so that it is spatially indistinguishable from Sgr A* itself. Spectrally, determining the relative velocity of the star to < 1 km/s precision enables scientists to discern the impact on its orbit of the black hole’s quadrupole moment, which according to general relativity is fully determined by the spin. Sufficient precision for this measurement can be reached via internal referencing between the stellar absorption features and the atmospheric absorption features imprinted into the observed continuum.

Massive Black Holes
An increasing effort to determine the masses of black holes in globular clusters has led to a variety of tantalizing results, but without robust detections. One of the key questions concerns the slope of the MBH – σ relation between the mass of the central black hole and the velocity dispersion of the stellar spheroid around it. Initial measurements of black holes in elliptical galaxies and classic bulges of disk galaxies had suggested MBH∝σ4. More recent assessments have argued in favor of a steeper slope of 5.6, which has implications on the physical processes underlying the relation. In contrast, a compilation of black hole limits for globular clusters concludes that the slope for those is closer to 2.3. This rather shallower slope would imply the relation to be defined by a process different to that in galaxies, perhaps suggesting that many of these systems are the stripped nuclei of dwarf galaxies.
At the moment, this issue is entirely open, and is unlikely to be resolved by currently available facilities. The problem is again the extreme crowding, which occurs in the centers of star clusters, exactly where measurements are needed for distinguishing scenarios with and without black holes. Proper motions, rather than just line-of-sight velocity dispersions, are required to measure and account for anisotropy, which can have a major impact on the black hole mass derived. Suitable measurements will only become possible with ELTs, when spatial resolution can overcome the crowding.

Exoplanet Characterization
Now that a large number of exoplanets are known, we are entering a phase driven by the need to characterize these planets, in particular the atmospheres of giant exoplanets. Direct imaging of exoplanets provides an opportunity to do this with intermediate band filters that cover molecular absorption bands, enabling us to distinguish models with different temperatures, surface gravities, and clouds. The large aperture of the ELT offers a multiple gain for such work: the small inner working angle, the increased contrast between the bright core and the speckles around it, and the elongation of the speckles when imaged through a broad or intermediate band (making them easier to distinguish from exoplanets). As such, the focus for MICADO will be in terms of exoplanets at small orbital separations (~ 1 astronomical units) around nearby stars (< 20 parsecs); exoplanets at larger separations (> 10 AU) around nearby stars as well as more distant stars (> 100 parsecs), and the circumstellar disks from which they form.
Figure 5 compares SPHERE observations of the planetary system around HR 8799 to simulations for MICADO, providing a glimpse of what it may be capable of achieving. The central panel shows a coronagraphic simulation of how this system might appear with MICADO after adding 30 seconds of exposures. The elongated central region is the effect of the wind on the residual halo close to the suppressed PSF core. The cleaned control region is clearly visible, as is its hexagonal boundary. Even in this raw image, the inner two planets can already be seen. The right panel shows that, after basic processing, one is in principle able to see fainter planets closer in. Two giant planets have been added, at 10 AU and 5 AU. By imaging these through various intermediate band filters, it is possible to estimate their temperature (700 K and 1,300 K respectively) due to their different molecular absorption properties. This opens up the exciting possibility that MICADO will be able to directly image planets for which a mass estimation is available from Gaia.
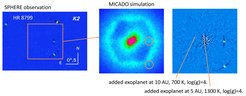