KMOS3D
Resolved Views of Galaxy Evolution at Cosmic Noon
The survey and science highlights below are largely taken from the ESO Messenger article about KMOS3D.
Our KMOS3D survey of Hα+[NII]+[SII] line emission of galaxies at redshift z ~ 0.7–2.7 exploited the powerful and efficient near-infrared (near-IR) multi-IFU instrument KMOS at the ESO VLT to investigate galaxy evolution. With deep observations of 740 targets spanning over two orders of magnitude in galaxy mass, five billion years of cosmic time, and all levels of star formation, KMOS3D provides an unparalleled populationwide census of spatially resolved kinematics, star formation, outflows, and nebular gas conditions. Selected highlights are summarized below. For a full list of articles featuring KMOS3D results, see the Publications section.
The Role of Near-IR IFU Surveys and KMOS3D
Spatially and spectrally resolved information on the kinematics, star formation, and ISM conditions on subgalactic scales provides insights into the physics that drive the early evolution of galaxies. Nebular emission lines such as Hα, [NII], [SII], [OIII], and Hβ are powerful tools for investigating these properties. At z ~ 1–3, the peak epoch of cosmic star formation, these rest-optical lines are redshifted into the near-IR regime (λ ~ 1–2.5 μm) and strongly dimmed. Dissecting distant galaxies requires sensitive near-IR instrumentation and a large collecting area.
Building on our earlier studies with SINFONI at the VLT, we carried out KMOS3D, a comprehensive 75-night GTO survey of Hα+[NII]+[SII] emission of 740 mass-selected galaxies at z ~ 0.7–2.7 (Wisnioski et al. 2015). The cornerstones of the survey strategy were 1) a wide and homogeneous coverage in galaxy stellar mass, star formation rate (SFR), colors, and redshift, 2) the same spectral diagnostics across the entire redshift range, and 3) deep integrations to map faint, extended line emission.
The targets were drawn from the Hubble Space Telescope (HST) "3D-HST" Treasury Survey (e.g., Momcheva et al. 2016), providing a well-characterized parent sample with source detection and accurate redshifts relying on rest-optical properties, largely reducing the bias toward blue, actively star-forming galaxies of rest-UV identification. 3D-HST overlaps with the CANDELS fields, with high-resolution HST optical and near-IR imaging, and extensive X-ray to far-IR/radio coverage. The KMOS3D selection criteria were 1) M⁎ > 109 M☉ and a magnitude cut of KAB < 23 mag, 2) a redshift of 0.7 < z < 2.7, and 3) the emission lines of interest falling in atmospheric windows and away from bright sky lines. By avoiding selection based on colors or properties sensitive to star formation or AGN activity, and by covering five billion years of cosmic time, KMOS3D is optimally suited for population censuses and evolutionary studies.
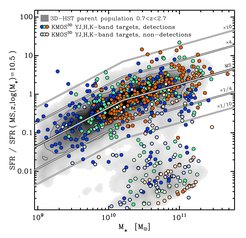
Figure 1 illustrates the distribution of the KMOS3D sample in stellar mass vs. SFR. The K-band magnitude cut ensures a 90% completeness at log(M⁎/M☉) > 9.6 at 0.7 < z < 1.1, > 10.2 at 1.3 < z < 1.7, and > 10.6 at 1.9 < z < 2.7 — these correspond to the redshift slices where Hα is observed in the Y-, J-, H-, and K-bands, respectively. The corresponding median on-source integration times are 5, 8, and 9 hours, and range up to 20 to 30 hours. The median angular resolution is 0.5 arcseconds, or ~ 4 kiloparsecs at z ~ 0.7–2.7. Down to SFRs about seven times below the "main sequence" (MS) of star-forming galaxies (comprising 4/5 of the sample), 90% of the objects are detected in Hα and 85% of them are resolved. Unsurprisingly, the detection rate drops among more quiescent galaxies, but still ~ 25% of them are detected, and 1/3 of these are resolved.
The Prevalence of Rotationally Supported Turbulent Gas-Rich Disks
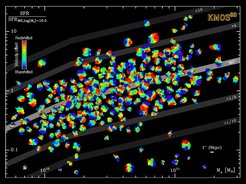
First and foremost, the spatially resolved gas motions derived from the Hα emission line profile across the KMOS3D galaxies robustly confirmed that the majority (> 70%) of high-redshift star-forming galaxies are rotating disks (Figure 2). The data also confirmed the elevated gas turbulence of high-redshift disks, with typical intrinsic disk velocity dispersions of σ0 ~ 50 km/s at z ~ 2.3 and ~ 30 km/s at z ~ 0.9 (Figure 3; Übler et al. 2019). The increase with redshift in the velocity dispersions is in line with expectations for gravity-driven turbulence in marginally stable gas disks and the cosmic evolution in gas fractions, where heating is caused by rapid gas flows onto and within the galaxies (e.g., Genzel et al. 2011; Krumholz et al. 2018).
Outer Disk Kinematics and the Dominance of Baryons
KMOS3D strengthened the key finding from smaller samples that z ~ 1–2.5 star-forming galaxies are not only gas-rich but also strongly baryon-dominated on ~ 10–15 kiloparsec scales (Figure 3). Kinematic modeling of 240 well-resolved disks with high S/N data showed that gas and stars dominate the total dynamical mass budget, making up ~ 55% on average and reaching ~ 90% at z ~ 2.3, leaving little room for dark matter within the disk’s half-light radius R1/2 at z > 2 (Wuyts et al. 2016). However, the scatter in baryonic mass fractions at fixed redshift is large due to correlations with mass surface densities. These effects are reflected in the evolution of the Tully-Fisher relation, connecting the disk circular velocity and mass, derived from KMOS3D (Übler et al. 2017). A combination of three factors can explain the trends in mass budget and Tully-Fisher relation: the gas and baryonic fractions of the disks increase with look-back time as enhanced cosmic accretion promotes high baryon concentrations at the center of dark matter halos, these halos have shallow profiles, and more compact disks probe less far into their host dark matter halo.
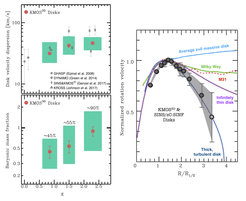
Recent exploration of the outer rotation curves of high-redshift disks led to the exciting discovery of steep declines in velocity beyond a peak at radius r ~ 1.3×R1/2, in stark contrast to the situation at low redshift. In very deep SINFONI observations of six high-mass star-forming galaxies probing out to 2–3×R1/2, the slopes are as steep or steeper than the Keplerian decline (vrot ∝ R1/2) for a disk purely supported by rotation, and detailed modeling implies dark matter fractions of ≤ 20% within a half-light radius (Genzel et al. 2017). KMOS3D substantiated this breakthrough by showing, through a novel stacking technique, that dropping outer rotation curves to 3–4×R1/2 are likely to be a widespread feature of log(M⁎/M☉) ≥ 9.5 star-forming disks around cosmic noon (Lang et al. 2017). In local spiral galaxies, the typically flat rotation curves are the hallmark of dominant dark matter in outer disk regions. At high redshift, however, the dominance of baryons within the shallower inner dark matter potential leads to declining rotation curves, with the considerable dynamical support from random motions further steepening the falloff. These results support the less important role of dark matter on disk scales implied by the mass budget and Tully-Fisher studies, independently of assumptions on the light to baryonic mass conversions. These findings also link massive z ~ 1–3 star-forming galaxies to their descendants — high-mass early-type galaxies and strongly bulged disks at z ~ 0 — which also have low central dark matter fractions.
Galactic Outflows – Demographics and Physical Properties
In rest-optical nebular line emission, outflows manifest themselves through a broad component underneath a narrower component from star formation, whose separation is facilitated with IFU data. Outflows driven by star formation (SF) are characterized by broad emission with FWHM ~ 400–500 km/s, detected across the disks and typically associated with brighter star-forming clumps. Faster winds (FWHM ~ 1,000–2,000 km/s) originate from the nuclear regions in the most massive galaxies hosting an AGN. An analysis of Hα+[NII]+[SII] emission of ~ 600 galaxies spanning 9 < log(M⁎/M☉) < 11.7, mostly from KMOS3D, revealed the distinct demographics and physical properties of outflows (Figure 4; Genzel et al. 2014; Förster Schreiber et al. 2019). The prevalence of SF-driven outflows depends on SF properties with fractions increasing above the MS and at SFR surface densities > 1 M⊙ yr-1 kpc-2, reaching up to ~ 40%. In contrast, AGN-driven outflows are rare below log(M⁎/M☉) ~ 10.7 and rapidly become ubiquitous above this mass, being present in ~ 75% of log(M⁎/M☉) > 11.2 galaxies. Similarly to AGN, their incidence depends primarily on stellar mass and its central concentration but is higher than that of AGN identified by any one diagnostic alone (e.g., X-ray properties), reflecting the variability of the AGN phenomenon in different tracers.
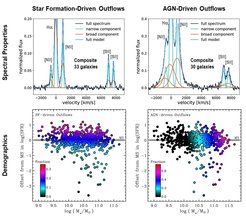
The velocity of SF-driven winds varies little with galaxy mass, implying that the bulk of expelled gas escapes only from log(M⁎/M☉) ≲ 10.3 systems while more massive galaxies drive so-called "fountains." Surprisingly, the inferred mass outflow rates Ṁout are only ~ 0.1–0.2 times the SFRs at all galaxy masses, at odds with theoretical work that requires mass loading factors η = Ṁout/SFR above unity and ∝(1/M⁎)0.35–0.80 to reproduce the observed slope of the galaxy mass – metallicity and galaxy mass – halo mass relationships at log(M⁎/M☉) < 10.7. This tension could be alleviated if substantial amounts of mass, momentum, and energy were contained in much hotter and/or colder wind phases than the ~ 104 K ionized gas probed by Hα, as inferred in nearby starburst winds.
The faster, high-duty-cycle AGN-driven winds at high masses have comparable mass loading factors to the SF-driven winds but carry ~ 10 times more momentum and ~ 50 times more energy, so that they can escape the galaxies, contribute to heat halo gas, and help prevent further gas infall. Numerical simulations suggest that such a mechanism, acting also at the modest AGN luminosities and Eddington ratios of a majority of the KMOS3D AGN, may be more effective at widespread and long-term quenching than ejective “QSO mode” feedback in rare, high-luminosity, high-Eddington-ratio AGN. The sharp increase in incidence of AGN-driven outflows near the transition mass above which passive galaxies become prevalent and both galactic molecular gas fractions and specific SFRs drop steeply strengthens the notion of a causal link between AGN activity and quenching.
Rejuvenation of Star-Forming Disks in Compact Galaxies
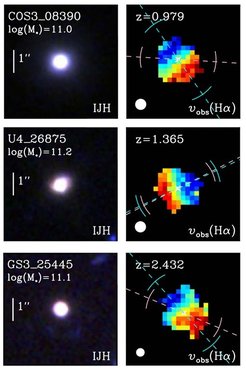
A strength of the KMOS3D strategy is to allow a systematic exploration of the emission line properties in rarer subsets of galaxies, with consistent comparisons with the underlying population. Massive compact star-forming galaxies at z ~ 1–3 have received much attention in recent years as potential immediate progenitors of dense massive quiescent galaxies. In 2/3 of 35 compact star-forming galaxies observed in KMOS3D, spatially resolved line emission reveals rotating gas disks with up to twice the extent of the compact stellar cores (Figure 5; Wisnioski et al. 2018). Their kinematic properties are similar to those of mass-matched, more extended star-forming galaxies in KMOS3D. They host AGN 1.4 times more often, in agreement with other recent studies, and they commonly drive powerful nuclear outflows. The rotation observed in the compact star-forming galaxies, and the growing evidence from morphologies and stellar kinematics that high-redshift passive galaxies are disk-like systems, support this evolutionary link.
Pushing into a regime so far unexplored with IFUs at z > 1, line emission was also detected in ~ 1/5 of the KMOS3D targets classified as quiescent based on their rest-frame colors (Belli et al. 2017). Half of them exhibit spectral signatures revealing the persistence of AGN activity and gas outflows well into quenching. In the other sources, the Hα luminosities confirm the low star formation activity with SFR(Hα)~0.2–7 M⊙ yr -1 but, surprisingly, their average [NII]/Hα ratio indicates about three times lower metallicities than MS star-forming galaxies of the same mass, and half also exhibit resolved gas disks. These properties suggest rejuvenation, where the low-level star formation is fueled by recent accretion of metal-poor gas via cosmic flows or minor mergers rather than being associated with advanced stages of disk fading.
References:
Belli et al. 2017, ApJ, 841, L6
Förster Schreiber et al. 2019, ApJ, 875, 21
Genzel et al. 2011, ApJ, 733, 101
Genzel et al. 2014, ApJ, 796, 7
Genzel et al. 2017, Nature, 543, 397
Krumholz et al. 2018, MNRAS, 477, 2716
Lang et al. 2017, ApJ, 840, 92
Momcheva et al. 2016, ApJS, 255, 27
Übler et al. 2017, ApJ, 842, 121
Übler et al. 2019, ApJ, in press (arXiv:1906.02737)
Wisnioski et al. 2015, ApJ, 799, 209
Wisnioski et al. 2018, ApJ, 855, 97
Wuyts et al. 2016, ApJ, 831, 149